The intention of this task is to assess the codes currently in use globally for analysis of wave energy devices. This effort will focus on assessing the accuracy and validation process of the codes by comparing codes to codes and codes to experiments.
The validation will focus on performance, loads, and related responses for a single devices and arrays of devices operating in defined wave conditions
The objectives are the following:
- To assess the accuracy and establish confidence in the use of numerical models.
- To validate a ranges of existing computational modeling tools
- Identify simulation methodologies leading to:
- Reduce risk in technology development
- Improved device energy capture estimates (IEC TC 102
- Improved loads estimates
- Reducing uncertainty in LCOE models
- Future research and development needed to improve the computational tools and methods
Milestones
The relevant work milestones are outlined as follows:
M 1 Develop a detailed work plans for the wave simulation model verification and validation.
M 2 Preform a review of available theoretical solutions for special cases that can be used for baseline verification of computational modeling tools and experimental model scale verification.
M 3 Perform simulations to develop data for a code-to-code comparison that gives a side-by-side comparison of the simulation results.
M 4 Perform simulations to develop code-to-experiment comparisons that give a side-by-side comparison of the simulation results.
Sub-tasks
Thework plan will include, but not be limited to the following:
- Define verification strategies and validation practice to be used.
- Compare analytical, experimental, and numerical results.
- Developing and identifying suitable analytical models of wave energy converters for verification of the numerical models.
- Developing or identifying base line test cases that can be used for a first comparison between simulations in relation to a limited suite of experimental test cases for validation based on available experimental data. Selecting a limited number of test cases and performing simulations.
- Analyze the simulation results based on the metrics. Develop a simple comparison charts for validation purposes. Compare and evaluate the numerical simulation results in a side-by-side fashion and compare and evaluate the simulation results with respect to experimental data in a side-by-side fashion using the metrics developed.
- Discussing the need, practicality and cost of obtaining additional experimental test data for model validation and making written recommendations to the ExCo and the technical community.
- Prepare annual progress reports in power point form for the ExCo meetings.
- Prepare technical reports and papers on the results in the public domain at conferences and in peer reviewed journals.
The first test case investigated under this Task has been a very simple test case simulating the heave decay of a sphere in still water from an initial offset. This was followed by simulations of the sphere in regular and irregular waves and an imposed linear damping. The numerical results from the many partners involved in this Task were compared, and results were discussed and published at EWTEC 2017 and presented at the 3rd International Conference on Renewable Energies Offshore in October 2018 in Lisbon. During 2018, the team investigated how existing experimental test data from the US Navy MASK basin for a heaving buoy could be used to validate numerical results.
A complete overview of the work done on heaving bodies was published in September 2019 in the Journal of Marine Science and Engineering: OES Wave Energy Modelling Task: Modelling, Verification and Validation of Wave Energy.
In January 2019, the work on simulating the MASK Basin experiments was completed and presented at a webinar. The options for follow up tasks were discussed, and it was proposed to simulate an Oscillating Water Column (OWC) system. Two sets of existing OWC data were presented and discussed:
- ??data from a small 1:50 scale OWC model which had been tested at DTU in Denmark;
- data from a larger 1:4 scale OWC model tested at KRISO in Korea. The choice was made to use the KRISO experimental data.
A simulation plan of the “KRISO test case” was presented in May. It was agreed that the CFD model team should focus only on a few wave cases, while the group using linear or weakly nonlinear models would simulate all the tests cases. KRISO uploaded the experimental data to the NREL share point during the summer of 2019.
NREL presented their simulation results at the 3rd webinar in September and WAMIT data was supplied by DTU for all to use. The numerical results compared relatively well, but discrepancy was noted, and it was decided to meet face to face in Amsterdam at the end of November 2019. At this meeting the results from both linear and CFD models were presented and the differences between simulated and experimental results were discussed. It was decided to incorporate the effect of air compressibility in the OWC to investigate which impact this would have on the simulation results. At the workshop, a set of dedicated experimental sphere decay tests from AAU was also presented, with the proposal to use these data to compare with the initial theoretical results. The workshop was supported by the OES and by the European funded project WECANET.
Public dataset from heave decay tests of a floating sphere
Highly accurate and precise heave decay tests on a sphere with a diameter of 300 mm were completed in a meticulously designed test setup in the wave basin in the Ocean and Coastal Engineering Laboratory at Aalborg University, Denmark.
The tests were dedicated to providing a rigorous benchmark dataset for numerical model validation, and thus a thorough uncertainty analysis was carried out to the test results. The study includes a comparison of the physical test results to the results from several independent numerical models based on linear potential flow, fully nonlinear potential flow, and the Reynolds-averaged Navier–Stokes (RANS) equations.
The physical test results are very suitable for numerical model validation and are public as a benchmark dataset. A paper with detailed descriptions of the test setup and findings have been published in the special issue Wave Energy System Hydrodynamics Modeling and Application of High-Performance Computing of the Energies journal.
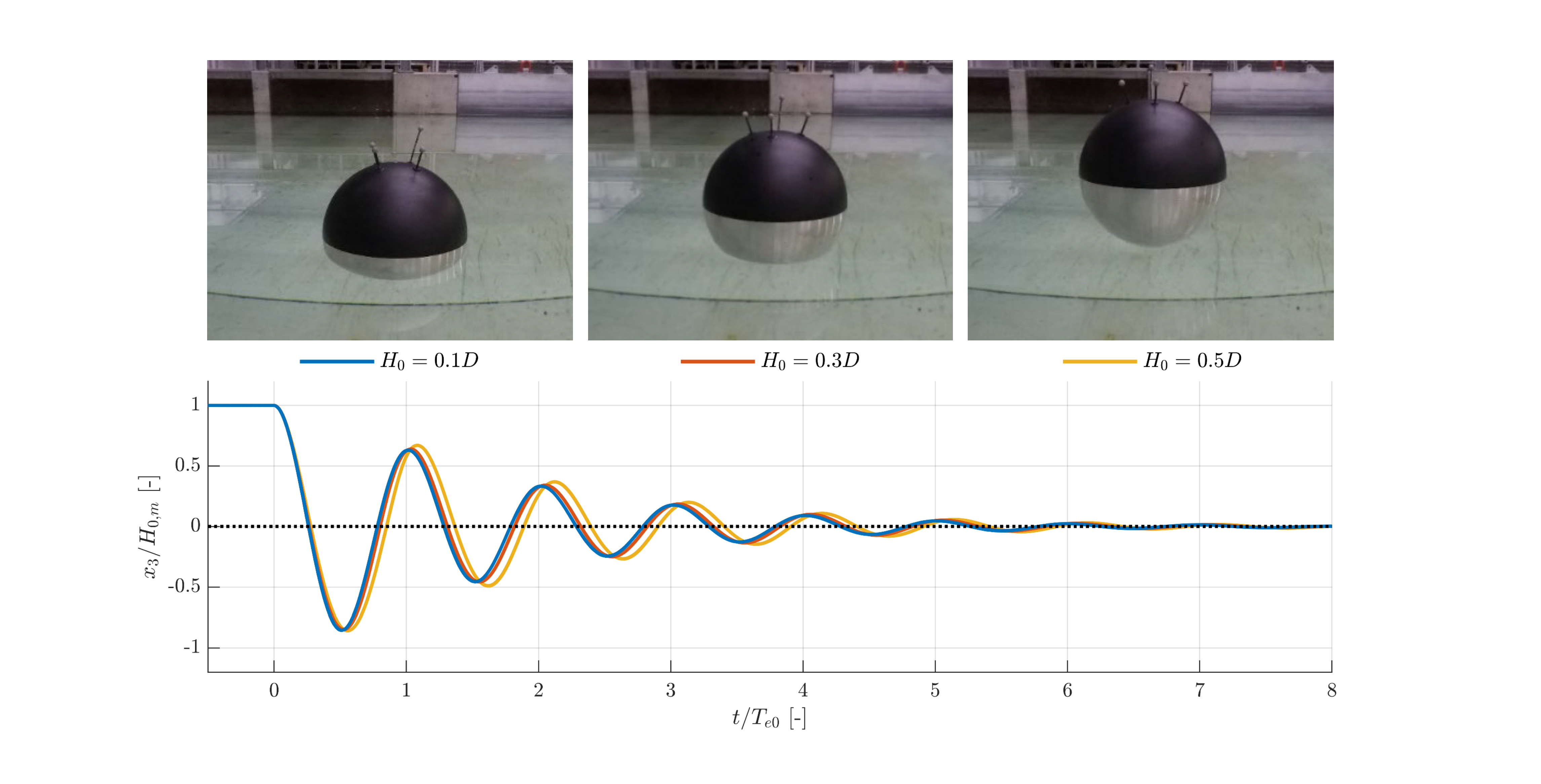
The benchmark dataset of the physical heave decay test is available on the file below (datafile.zip). In addition all numerical modelling blind tests of the text case are also available.